Hydrothermal
Contents
Hydrothermal Vents
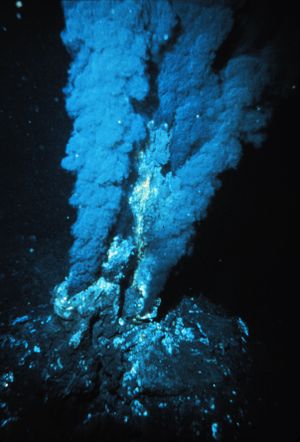
Hydrothermal vents typically spew out superheated fluid as high as 407 degrees Celsius at velocities ranging from 1-5 m/s. The vent openings are anywhere from a couple inches to several meters. The 1-5 m/s flow happens at depths of typically 2,300 m below sea level which is under pressure of about 200 atmospheres. The vents are powered by the weight of seawater above forcing itself into fissures within the Earth's crust which is then returned to the ocean as a continuous, superheated geyser.
Marshall Hydrothermal Recovery System
The Marshall Hydrothermal Recovery System is the first patented system designed to utilize underwater hydrothermal vents for energy, mining, and water desalination. It was designed by Bruce C. Marshall of California.
In one proposed embodiment of the system, the vent would be capped off and the hydrothermal fluid ducted to the sea surface through highly insulated pipes. A floating platform or ship on the surface would then extract the heat out of the hydrothermal fluid and then use it for power generation.
In the closed loop system embodiment, a loop of insulated pipe would go from a floating platform or ship on the surface, down to the ocean floor, next to or in the hydrothermal vent, and would return to the ship or platform. A heat exchanger placed within the hydrothermal fluid flow would heat working fluid which would then be directed to the surface and used for power generation. The used working fluid would then be returned to the sea floor and be reheated.
Recoverable Energy
A 3m opening and 3 m/s flow at 350 degrees Celsius would create a flow of 21,205.73 L/sec, which translates into 29,298.77 MW of raw power, calculated as the reciprocal of the amount of energy needed to raise that volume of water to that temperature. In comparison, the largest fission nuclear reactor in the U.S. provides 4,000 MW of power.
Computer modeling has shown the estimated producible energy after generator losses to be about 1 MW/10 cm2 pipe area.
Advantages of Hydrothermal Energy over Other Alternative Sources
Hydrothermal energy is by far the densest and most highly concentrated natural source. Its energy content is estimated to be about 3.3x106 more intense than solar radiation, and it is available 24 hours a day. By contrast, both wind and solar suffer from low density and intermittent operation. Hydrothermal energy offers the potential of replacing existing power plants, something that other renewable sources can not promise.
Deep-Sea Mining
When using the open-loop configuration, the Marshall Hydrothermal Recovery System proposes to capture the ores that are being ejected from the core of the Earth before they can settle to become hydrothermal veins. Hydrothermal veins (hydrothermal vents of the geologic past) are the natural source of virtually all surface mines in the world. The ores are among the richest ever harvested, and because of the huge variety of metals and minerals in the fluid, mining promises to be as valuable as, or perhaps even more valuable than the energy that will be produced.
Water Desalination
In the open-loop configuration, the Marshall Hydrothermal Recovery System allows the water component of the hydrothermal fluid to flash to steam, which can then be distilled and recovered as fresh water. The vast majority of the energy needed is provided by nature, but additional stages of purification may be needed.
Technical challenges
The depth of the hydrothermal vents at Juan de Fuca plate is about 1500m, well within reach of conventional oil rigs. But other technical challenges remain.
For the open system: if a vent was successfully capped, would the hydrothermal fluid continue flowing? Would the heat rise so that it can be used at sea level?
For the closed system: how much heat could be captured from the vent using a heat exchanger?
Subsea construction is always difficult, and those who would build the first Marshall Hydrothermal Recovery System will have to solve major primary engineering challenges on a scale of those faced when building the world’s first nuclear power plant. Materials and procedures must be utilized to deal with the highly acidic (and in some cases highly alkaline) vent fluid, and selecting the best method of exchanging the heat and the best working fluid will be an immediate concern.
Submarine cable is costly and has never been laid at the depths in question. The main problem is not the depth as one might assume, but the tensile strength of the cable. The weight of such a great length of cable is sufficient to tear it in half during the laying process if it is not properly designed. The most economical means of recovering the ores from the mining products must also be determined.
Economic challenges
How much would either system cost? Would either be competitive with traditional methods of power generation such as nuclear?
A nuclear power plant costs 2000 per kW so a 4GW plant would cost about $8 billion.
A semi-submersible oil platform for deep ocean use costs $500 million to $1 billion. Drilling the vent would cost 100 million (probably less because one would not need to drill deep into the earth).
An undersea cable from Juan de Fuca would have to be 200 miles which is around the same length of the longest underwater cable. Unfortunately, transmission capacity for that cable is only 700 MW and it cost 550 million euros which is about 800 million dollars. So to reach 4 GW 6 of these cables would be needed which is $4.8 billion. Total so far, about $5.8 billion and the generators haven't been accounted for.
From these estimates, this proposal is definately viable but there are many technological hurdles that need to be considered. Even with the engineering challenges the system faces, it stands to be extremely profitable because of the triple revenue streams of energy, mining products, and water, and the lack of external fuel.